Inside the Wake Forest Institute for Regenerative Medicine, more than 300 scientists in the fields of biomedical and chemical engineering, cell and molecular biology, biochemistry, pharmacology, physiology, materials science, nanotechnology, genomics, proteomics, surgery and medicine work side by side to translate the science of regenerative medicine into clinical therapies.
It All Starts with Cells
Engineering an organ or tissue begins with having the right kinds of cells. In some cases, cells are isolated from a small tissue sample the size of a postage stamp. They are then mixed with growth factors and multiplied in the lab. The cells multiply in quantity so rapidly that, in about 6 weeks, a layer one cell thick could theoretically cover a football field.
For cell types that cannot be adequately grown outside the body (like heart, nerve, liver and pancreas cells, for example), stem cells may be an option because of their ability to become multiple cell types. Scientists in our lab identified a new source of stem cells -- amniotic fluid and placental tissue. These cells are readily obtainable and, unlike embryonic stem cells, do not form tumors. We are currently using the cells to explore potential treatments for diabetes and for liver and heart disease.
These images show muscle precursor cells in culture. When differentiated, the cells become muscle fibers (the “streaks” in the photo on the right).
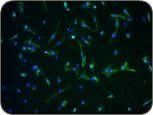
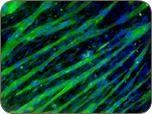
Making a Scaffold
After cell expansion, the next step in engineering a tissue or organ is to create a mold, or scaffold, in the shape of the tissue. Electrospinning is one technique used to make scaffolds for blood vessels, as well as muscles and tendons. The technique involves dissolving a biomaterial into a solvent, loading it into a syringe, and then applying a high voltage to the solution as it is slowly ejected from the syringe.
Watch a video example of electrospinning below:
At some distance away, there is a collection mandrel that is grounded so that when the charged solution leaves the syringe, it is attracted to the mandrel. You can actually see in this video the fibers shooting from the syringe to the mandrel and forming a tubular structure.